|
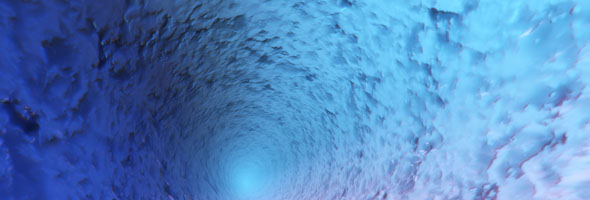 |
 |
 |
|
Prehistory: Suprachiasmatic nucleus
is a paired cute ovoidal nuclei displaying robust circadian
oscillation |
Okamura-lab starts in 1995 in Kobe, when Hitoshi Okamura
becomes a professor of the Department of Anatomy II in
Kobe University School of Medicine. Thereafter, main
theme is the molecular mechanisms of mammalian circadian
clock. Before describing the achievements of the laboratory,
its prehistory is described briefly.
In the Department of Anatomy II in Kyoto Prefetural University
of Medicine (Professor Yasuhiko
Ibata), Okamura encountered
the dense cluster of vasoactive intestinal peptide (VIP)
producing neurons symmetrically located just dorsal to
the optic chiasma, which strongly impressed the dignity
of the suprachiasmatic nucleus (SCN) at 1983. At that
time, we analyze SCN by histochemical and electron microscopic
techniques. During these days, we had a great effort
to established two completely original methods which
will be fruitful in later days. The first is the highly
quantitative histochemistry (Brain Research, 1987; Mol
Brain Res, 1995; J. Neuroscience, 1997), and the second
is the in vitro organotypic slice culture technique for
the study of the SCN (Neuroscience, 1994: collaboration
with Professor Shin-ichi Inouye). At this time, we did
not notice its powerfulness, but both two are flowered
after 10 years when mPer genes are discovered.
In France in Lyon and Gif-sur-Yvette supported by INSERM
and CNRS (1987-1989)(Professors Michel
Jouvet, Robert Naquet), we found that virtually all SCN neurons are
GABAergic.
|
1) |
Takahashi Y, Okamura
H, Yanaihara N, Hamada S, Fujita S, Ibata Y: Vasoactive
intestinal peptide immunoreactive neurons in the
rat suprachiasmatic nucleus demonstrate diurnal variation.
Brain Res., 497: 374-377, 1989. |
2) |
Okamura H, Abitbol M, Julien J.F,
Dumas S, Berod A, Geffard M, Kitahama K, Bobillier
P, Mallet J, Wiklund L: Neurons containing messenger
RNA encoding glutamic acid decarboxylase (GAD) in
rat hypothalamus demonstrated by in situ hybridization,
with special enphasis on cell groups in medial preoptic
area, anterior hypothalamic area and dorsomedial
hypothalamic nucleus. Neuroscience, 39: 675-699,
1990. |
3) |
Tominaga K, Inouye S -I T, Okamura
H: Organotypic slice culture of the rat suprachiasmatic
nucleus: Sustenance of cellular architecture and
circadian rhythm. Neuroscience, 59: 1025-1042, 1994. |
4) |
Okamura H, Kawakami F, Tamada Y,
Geffard M, Nishiwaki T, Ibata Y, Inouye S-IT: Circadian
change of VIP mRNA in the rat suprachiasmatic nucleus
following p-chlorophenylalanine (PCPA) treatment
in constant darkness. Mol. Brain
Res., 29: 358-364,
1995. |
5) |
Ban Y, Shigeyoshi Y, Okamura H:
Development of circadian VIP rhythm in the rat suprachiasmatic
nucleus. J. Neurosci., 17, 3920-3931, 1997. |
|
|
Although the circadian clock genes
were identified in 1984 in Drosophila, mammalian molecular
mechanism of circadian rhythms was totally unknown even
at 1995. For example, there were many groups believing
the circadian rhythms being produced by the networks
of neurons as sleep-wake cycles, since many molecular
efforts during 13 years had failed to isolate the clock
genes in mammals. In 1997, we discovered the human and
mouse genes encoding PAS-domain (PAS, a dimerization
domain present in Per, Amt and Sim)-containing polypeptides
that are highly homologous to Drosophila period by the
collaboration of Dr. Hajime Tei in Tokyo University (Nature,
1977). We named this gene as mPer1, and thereafter, we
proceeded the molecular dissection solely in my laboratory,
and isolated another two mammalian mPer genes (mPer2 and mPer3) and mammalian homology of timeless (mTim)
(EMBO-J, 1998). Subsequently, we have found that mPer1 is light-inducible and acts as a “pendulum”, committing
the phase-shift of the circadian clock by light (Cell,
1997). Collaborating with Professor Jay
Dunlap (Dartmouth
University), we found that mammalian clock is a day-type
clock similar to Neurosopra, although Drosophila’s clock
is night-type clock. Together with the discovery of Clock gene by Professor J. Takahashi, our studies contributed
the establishment of the concept that the mammalian circadian
rhythms are generated at the gene level.
|
1) |
Tei H, Okamura H, Shigeyoshi
Y, Fukuhara C, Ozawa R, Hirose M, Sakaki Y: Circadian
oscillation of a mammalian homologue of the Drosophila
period gene. Nature 389, 512-516, 1997 |
2) |
Shigeyoshi Y, Taguchi K, Yamamoto
S, Takekida S, Yan L, Tei H, Moriya T, Shibata S,
Loros JL, Dunlap JC, Okamura H: Light-induced resetting
of a mammalian circadian clock is associated with
rapid induction of the mPerl transcript. Cell 91,
1043-1053, 1997. |
3) |
Takumi T, Taguchi K, Miyake S, Sakakida
Y, Takashima N, Matsubara C, Maebayashi Y, Okumura
K, Takekida S, Yamamoto S, Yagita K, Yan L, Young
ML, Okamura H: A light independent oscillatory gene
mPer3 in mouse SCN and OVLT. EMBO
J. 17: 4753-4759,
1998. |
|
|
Fig. 1 Clock genes and a model of the
core feedback loop in the mammalian cells. |
|
 |
Although we are interested in producing knockout mice
of these mPer genes to characterize these genes, we have
neither facilities to make these animals, nor financial
support at that time. So, we were searching low cost but
scientifically important works to prove that mPer genes
really have the clock oscillating ability. It is noteworthy
that the molecular studies suggest that mammals and Drosophila
utilize similar components to generate circadian (~24 hour)
rhythms. To prove that the mPer1 and mPer2 genes indeed
have the capability to generate oscillations, we collaborated
with Professor Amita Sehgal (University of Pennsylvania)
the discoverer of Drosophila timeless, and investigated
whether the introduction of mouse mPer1 and mPer2 genes
into the arrhythmic per01 mutant of Drosophila caused functional
recovery. Behavioral assays showed that both mPer1 and
mPer2 constructs driven by Drosophila timeless promoter
restored rhythms in per01 flies that are otherwise arrhythmic
due to a lack of endogenous PERIOD protein. Thus, incorporation
of mammalian mPer1 and mPer2 genes into Drosophila mutants
demonstrates that both mPer1 and mPer2 can function as
clock components. This study is unique, and indeed appreciated
from some researchers, but the recovery rate of flies are
at most 20%, thus cannot be published in leading journals
at 1999 (Later we published the results at 2002 to Genes
to Cells). Later mice homozygous for the targeted allele
of mPer1 and/or mPer2 were reported by 3 groups (Drs. Lee,
Reppert and Sassone-Corsi), showing the severely disrupted
locomotor activity rhythms during extended exposure to
constant darkness. |
1) |
Shigeyoshi Y, Meyer-Bernstein
E, Yagita K, Weili F, Chen Y, Takumi T, Schotland
P, Sehgal A, Okamura H: Restration of circadian behavioral
rhythms in a period null Drosophila mutant (per01)
by mammalian period homologues mPer1 and mPer2. Genes
Cells, 7, 163-171, 2002. |
|
|
Fig. 2 Restoration of circadian rhythm in per01 flies
after the introduction of mammalian mPer1 or mPer2 genes.
A Actogram (left) and its period length analysis (right;
period length is indicated in hours at the top of periodigrams)
of transgenic flies (dtim-mPer1, dtim-mPer2) and controls
(per01 and wild type, WT). Circadian time is indicated
at the top of actograms, consecutive days at left of actograms.
The significance line denotes a 0.05 level of significance.
B Frequency distribution of activity periods in tim-mPer1- and tim-mPer2-containing transgenic flies. C Difference
of the period length in mPer1- and mPer2-
restored rhythms. From Genes Cells 7, 163-171, 2002. |
|
|
Just after the discovery of mPer genes, the mammalian
clock study goes Drosophila as a model. In this line, mTim,
mmammalian counter part of Drosophila
timeless, was soon
be cloned. Soon, clock and bmal1 are established as positive
transcription factors on mPer genes following the negative
feedback theory of Drosophila clock machinery. However,
the breakthrough comes from the unexpected field.
Professor Gijsbertus van der Horst, a researcher of DNA
repair in Netherlands, made the knockout mice of mammalian
cryptochromes (Cry1, Cry2), and surprisingly found that
these mice were completely arrhythmic in behavior (Nature,
1999).
Just after they got this result, we began the collaboration
whether master clock in the SCN are working or not. Okamura
and lab members trip to Rotterdam for one week experience:
fixed smples from these mice are successfully depicted.
By the quantitative in situ hybridization methods, we found
that the mPer gene oscillation was completely abolished
in the central clock (SCN) (Science, 1999).
At the same time, clock gene oscillation was abolished
in peripheral tissues (liver) of Cry deficient mice. Then
one question arises: does the peripheral clock oscillation
influence the behavioral arrhythmicity? We performed the
transplantation of wild-type SCN to arrhythmic Cry-deficient
mice, and found that the behavioral arhhythmicity was recovered
by the transplantation of wild-type SCN (Current Biology,
2003). This rhythm emergence strongly suggests the unnessessity
of non-SCN peripheral clocks for formation of behavioral
rhythms. This result claims the SCN as the synchronizer,
but strongly supports the SCN as the rhythm generator at
behavioral rhythms, although rhythms of peripheral clocks
(endocrine, or enzyme etc) have not been addressed. abolished
it. |
1) |
Okamura H, Miyake S, Sumi
Y, Yamaguchi S, Yasui A, Muijtjens M, Hoeijmakers
JHJ, van der Horst GTJ: Photic induction of mPer1 and mPer2 in Cry-deficient mice lacking a biological
clock. Science 286, 2531-2534, 1999. |
2) |
Sujino M, Matsumoto K, Yamaguchi
S, van der Horst G, Okamura H*, Inouye SIT*: Suprachiasmatic
nucleus grafts restore circadian behavioral rhythms
of genetically arrhythmic mice. Current
Biology 13,
664-668, 2003. (*Correspondence) |
|
|
Fig. 3 Double-plotted
locomotor activity rhythms of SCN-grafted Cry1/Cry2 double
knockout mice. Arrhythmic
Cry1/Cry2 double knockout mice first lesioned
SCN, then received brain transplantation operation of neonatal
SCN. Two weeks after transplantation, activity bouts gradually
consolidated to form a free-running rhythm with a shorter
than 24 hour period.
From Currrent Biology, 13, 6464-668, 2003. |
|
|
Since main components of core clock oscillation had been
revealed, a number of laboratories began to clarify the
molecular mechanisms of the mammalian clocks in 1998-2000.
The cellular circadian oscillation in mammals to start
with the transcription of two genes: mPer1 and mPer2. Expression
of these genes is stimulated by heterodimers formed by
the bHLH-PAS proteins CLOCK and BMAL1. We demonstrated
that mPER proteins made in the cytoplasm, translocate into
the nucleus (Genes Develop, 2000), and form a negative
complex comprised of mCRY1, mCRY2, mPER1, mPER2, mPER3
and mTIM that suppresses the transcription triggered by
CLOCK and BMAL1. Importance of posttranscriptional mechanisms
including phosphorylation and degradation are suggested.
We demonstrate that mPER1 and mPER2 proteins usually shuttle
between the cytoplasm and the nucleus and are easily degraded
by ubiquitination and the proteasome pathway (EMBO-J. 2002;
MCB, 2005). Ubiquitination of mPER proteins is inhibited
by the presence of mCRY proteins. Since mCRY protein can
also be ubiquitinated when mPER proteins are absent, the
mPER/mCRY dimer is stabilized against degradation, suppresses
mPer1 and mPer2 transcription, and shuts off mPER synthesis.
Since it is speculated that the transcription level of
mPer genes is determined essentially by the concentration
of mPER/mCRY dimer in the nucleus, re-starting mPer transcription
depends on the export of the mPER proteins out of the nucleus
by the CRM1/Exportin1 nuclear export machinery. The consequent
decrease of mPER destabilizes mCRY, and the decrease of
mCRY then allows mPer1 and mPer2 gene transcription to
restart. |
1) |
Yagita K, Yamaguchi
S, Tamanini F, van der Horst GTJ, Hoeijmakers JHJ,
Yasui A, Loros JJ, Dunlap JC, Okamura H: Dimerization
and nuclear entry of mPER proteins in mammalian
cells. Genes Develop. 14:1353-1363, 2000. |
2) |
Yagita K, Tamanini F, Yasuda M,
Hoeijimakers JHJ, van der Horst, GTJ, Okamura H:
Nucleocytoplasmic shuttling and mCRY dependent
inhibition of ubiquitination of the mPER2 clock
protein. EMBO J. 21, 1301ー1314, 2002. |
3) |
Yamamoto Y, Yagita Y, Okamura
H: Role of cyclic mPer2 expression in mammalian
cellular clock. Mol. Cell
Biol., 25, 1912-1921,
2005. |
|
|
Fig. 4 Phospholylation,
degradation and nuclear translocation of mPER proteins. |
|
|
The existence of cellular clock was indicated by the
demonstration of circadian expression of clock genes in
fibroblast cell lines after the serum-shock revealed by
Schibler’s group in Geneva. Are there any differences of
autonomously rhythmic clock genes in these cells and those
in the SCN? We addressed this question by using spontaneously
immortalized mouse embryonic fibroblasts (MEFs) from wildtype
and Cry1-/-Cry2-/- mice (Science, 2001). Both wildtype
and Cry1-/-Cry2-/- cell lines showed clock properties similar
to those found in the SCN of wildtype and Cry1-/-Cry2-/-
mice, respectively. These included: (i) temporal expression
profiles of all known clock genes, (ii) the phases of the
various mRNA rhythms (i.e. antiphase oscillation of Bmal1 and mPer
genes), (iii) the delay between maximum mRNA levels
and appearance of nuclear mPER1 and mPER2 protein, (iv)
the inability to produce oscillations in the absence of
functional mCry genes, and (v) the control of period length
by mCRY proteins. These results strongly support the conclusion
that the components and oscillatory mechanism of central
clocks (in the SCN) and of peripheral clocks are essentially
identical. |
1) |
Yagita K, Tamanini F,
van der Horst GTJ, Okamura H: Molecular mechanisms
of the biological clock in cultured fibroblasts.
Science 292, 278-292, 2001. |
|
|
Fig. 5 Endothelin-induced
time-dependent gene expressions of mRNA (black dotted
line) and proteins (red line) in mPer1 (A)
and mPer2 (B) in mouse embryonic
fibroblasts (MEF). Note 6-8 hours difference of mRNA and
proteins in both mPer1 and mPer2. (C)
(Left) Circadian expression of Per1, dbp and Bmal1 in wild
type MEFs and mCry1-/-mCry2-/- MEFs. (Right) mCry1-/-MEF
(square) and mCry2-/-MEFs (filled circle).From Science
292, 278-292, 2001. |
|
|
An important question is how the time information generated
by the cell clock oscillator is transmitted to the hundreds
of clock controlled genes (ccg) that represent the output
of the clock. Two routes are proposed: the first goes directly
from the central loop of the mammalian clock to the ccgs
through E-box (CACGTG). The second route is indirect, and
regulates the antagonistic effects of PAR proteins (DBP,
HLF, TEF) and E4BP4 on the D-box (Mol. Cell Biol., 2000;
Genes Develop 2001). PAR proteins activate the transcription
of target genes by binding to the specific sequence RTTAY
GTAAY (R, purine; Y, pyrimidine) during the day, and E4BP4
suppresses transcription of these target genes in the night.
E4BP4 and the PAR proteins may switch back and forth in
turning target gene transcription on and off. Since many
of genes contains D-box, it is speculated over 100 genes
are circadianly regulated by D-Box. |
1) |
Yamaguchi S, Mitsui
S, Yan L, Yagita K, Miyake S, Okamura H: Role of
DBP in the circadian oscillatory mechanism. Mol.
Cell. Biol. 20:4773-4781, 2000. |
2) |
Mitsui S, Yamaguchi S, Matsuo T,
Ishida Y, Okamura H: Antagonistic role of E4BP4
and PAR proteins in the circadian oscillatory mechanism.
Genes Develop. 15, 995-1006, 2001. |
|
|
Fig. 6 The core,
accessory and output molecular mechanisms of the mammalian
circadian clock. BMAL1/CLOCK heterodimer binds to E-box
in clock oscillating genes and ccg, and accelerates their
transcription. The core feedback loop provided by mPer1 and mPer2 is
supplemented by an accessory PAR protein loop. DBP accelerates
the mPer1 transcription,
but E4BP4 maximally expressed in counter phase to PAR
proteins suppresses mPer1 transcription.
From Cell Tiss Res 309, 47-56, 2002. |
|
|
Next, we will show the examples how core clock regulates
cellular functions by analyzing the association of circadian
clock and cell cycle. Since the life span of each cell
is limited, cell growth and mitosis are required to maintain
organ or tissue function. There is substantial evidence
that circadian rhythms affect the timing of cell divisions
in vivo: day-night variations in both the mitotic index
and DNA synthesis were found in oral mucosa, corneal epithelium,
and bone marrow. These studies used histochemical techniques
and normal physiological conditions, so the mitotic cells
comprised only a few per cent of the cells examined. A
suitable system with a high proportion of dividing cells
is required in order to apply biochemical techniques to
study the molecular links between regeneration and the
circadian clock. We noticed that the liver provides a suitable
organ since it is known to undergo vigorous regeneration
after incomplete surgical removal. Although the cell cycle
period overall is several months in unoperated animals,
a 2/3 partial hepatectomy (PH) induces the large majority
of the remaining, pre-existing hepatocytes to divide, and
the regeneration speed is so rapid that liver mass is restored
within 7days. Moreover, expression of mPer1 and mPer2 oscillates
vigorously in liver, and the temporal profiles and the
vigorousness of the expression of clock genes were not
altered after PH. Thus, the mouse liver is very suitable
for analyzing the molecular connection between the circadian
clock and the cell cycle.
We first compared the rate and timing of liver re-growth
after PH in mice, when PH was performed in the morning
at lights-on or in the afternoon (Science, 2003a). S-phase
kinetics, represented by the incorporation of bromodeoxyuridine
(BrdU), was similar in both morning-operated and afternoon-operated
animals. However, there was an 8 hour delay in the M-phase
(cells entering mitosis) when PH was performed at morning,
as compared to afternoon. This indicated that the timing
of the hepatectomy determines the timing of entry into
M-phase for these regenerating cells. To determine the
impact of circadian clocks on the cell cycle, clock-less
arrhythmic Cry1-/-Cry2-/- mice were subjected to PH (Science,
1999). In these mice, mitosis was severely impaired, and
liver regeneration was severely blunted.
We performed DNA arrays and Northern blots to characterize
the molecular differences in M-phase entry and found that
cyclin B1 and cdc2 were positively correlated, and wee1,
the gene for a kinase that inhibits mitosis by inactivating
CDC2/cyclin B, was negatively correlated to M-phase. This
is interesting since all these genes are cell cycle regulators
governing G2 to M transition, and these expression profiles
correlate well with M-phase progression. In the livers
of normal mice, there were clear circadian rhythms of wee1 expression, with very low levels in the morning and high
levels in the night. Levels of wee1 were always high in
Cry-deficient mice, whereas levels were always low in Clock mutant mice (Clock/Clock). There are three E-boxes in the
5' UPR of wee1, which were, furthermore, activated by CLOCK/BMAL1
and suppressed by PER2, PER3, CRY1 and CRY2. These results
suggest that wee1 transcription is regulated directly by
the core feedback loop through its E-box elements. Changes
in transcription of wee1 are reflected at the protein level,
influence CDC2 activity levels, and are negatively correlated
with the mitotic peak. WEE1 phosphorylates the cyclin-dependent
kinase CDC2, a key regulator of G2 to M transition. In
order to allow entry into mitosis, CDC2 has to be dephosphorylated
by CDC25, a protein phosphatase, and it is the competition
between the activities of WEE1 and CDC25 that determines
the phosphorylation status of CDC2 (and hence the propensity
of cells to enter mitosis). Only when WEE1 levels are low
(normally in the morning) can CDC25 phosphatase successfully
antagonize the action of WEE1. These findings, taken together,
demonstrate that the circadian clock controls the G2 to
S transition via the regulation of WEE1. Together with
the report demonstrating a high incidence of lymphoma in
mice lacking the mPer2, our present study sheds light on
the association of circadian rhythms and cell division. |
1) |
Matsuo T, Yamaguchi
S, Mitsui S, Emi A, Shimoda F, Okamura H: Control
mechanism of the circadian clock for timing of
cell division.
Science 302, 255-259, 2003. (published on line
in Science Express on 21 August 2003) |
|
|
Fig. 7 Control of
cell cycle by cell clock. Schematic representation showing
the link between the circadian clock and the cell cycle
(upper). Role of CDC2, cyclin, WEE1 and CDC25 in making
active MPF (M-phase promoting factor) is depicted at
the bottom. From J. Boil. Rhythms, 19, 388-399, 2004 |
|
|
Above studies revealed that cell clocks govern many of
the cellular functions. Then, is the cell-time transmitted
to another cells? We want to address this issue in the
SCN, since it is the sole organ oscillate eternally. We
adopt SCN-slice culture system which we established for
monitoring peptide-release (Neuroscience, 1994). We made
a SCN slice taken from transgenic mice carrying the luciferase
gene driven by the mPer1 promoter (mPer1-luc)
(Curr Biol, 2001). In this study, we discovered that the
application of NMDA, analogous to light stimuli to the
living animals, instantly altered the phase of the core
clock oscillation of slice-SCN phase-dependently. In collaborating
with Professor
Masaki Kobayashi, a specialist enabling the visualization
of very weak biophoton, we succeed to observe the rhythmic
transcription of genes at the single cell level by a cryogenic
high resolution CCD camera (Science, 2003). The SCN cells
showed robust transcription rhythms with a period length
of -24 hours, with several hundreds of cells expressing
mPer1 genes synchronously. Moreover, the individual oscillatory
cells are arranged topographically: the phase-leader with
a shorter period length is located in the dorsomedial periventricular
part of the SCN. A protein synthesis inhibitor (cycloheximide)
sets all the cell clocks to the same phase and, following
withdrawal, intrinsic interactions among cell clocks re-establish
the stable program of gene expression across the assemblage.
Tetrodotoxin, which blocks action potentials, not only
desynchronizes the cell population, but also suppresses
the level of clock gene expression, demonstrating that
neuronal network properties dependent on action potentials
play a dominant role in both establishing cellular synchrony
and maintaining spontaneous oscillations across the SCN.
Thus, the cell-rhythm oscillation generated by the core
clock oscillatory loop is coupled and amplified by the
ordered cell-cell communications in the SCN. |
1) |
Tominaga K, Inouye SI,
Okamura H: Organotypic slice culture of the rat
suprachiasmatic nucleus: sustenance of cellular
architecture and circadian rhythm. Neuroscience 59, 1025-1042, 1994. |
2) |
Asai M, Yamaguchi S, Isejima H,
Jounouchi M, Moriya T, Shibata S, Kobayashi M,
Okamura H: Visualization of mPer1 transcription
in vitro by luciferase mediated bioluminescence:
NMDA induces a rapid phase-shift of mPer1 gene
in cultured SCN. Current Biology 11, 1524-1527,
2001. |
3) |
Yamaguchi S, Isejima H, Matsuo T,
Ohkura R, Yagita K, Kobayashi K, Okamura H: Synchronization
of cellular clocks in the suprachiasmatic nucleus.
Science 302, 255-259, 2003. |
|
|
Fig. 8 Temporal changes
in bioluminescence signals from 100 SCN cells randomly
chosen in wild-type (upper figure) and mPer1-luc-bearing mCry1/mCry2 double-knockout
mice (lower figure). Note variety pattern of peaks in
cells of wild type animals, but total absence of rhythmic
cells in Cry double knockout mice.From Science 302, 255-259,
2003. |
|
|
By what route SCN regulates the peripheral clocks? Melatonin
regulates the sympathetic nervous system by directly acting
to the SCN (J. Physiol., 2003). More importantly, sympathetic
nervous system plays the key role. In collaborating with
Professor Shigenobu Shibata, we demonstrated that hepatic
gene expression of clock genes was regulated by the sympathetic
nerves (PNAS, 2003). Moreover, we demonstrated that SCN
regulates the circadian expression of adrenal corticosterone
by the activation of various genes by the route of innervating
sympathetic adrenal nerve (Cell Metabolism, 2005). Yes,
sympathetic nerve conveys the time of central clock to
peripheral organs, and the adrenal gland is the key organ
transforming circadian signals from nerve signals to the
endocrine signals. Released corticosterone may tune the
phase of peripheral clocks. |
1) |
Terazono H, Mutoh T,
Yamaguchi S, Kobayashi M, Akiyama M, Udo R, Ohdo
S, Okamura H, Shibata S: Adrenergic regulation
of clock gene expression in the mouse liver. Proc.
Natl. Acad Sci. USA 100, 6795-6800, 2003. |
2) |
Ishida A, Mutoh T, Ueyama T, Bando
H, Masubuchi S, Nakahara D, Tsujimoto G, Okamura
H: Light activates the adrenal gland: Timing of
gene expression and glucocorticoid release. Cell
Metabolism, 2, 297-307, 2005. |
|
|
Fig. 9 (Upper
figure) Light exposure induces the high level
of expression of luciferase luminescence in the adrenal
gland (arrows) in mPer1-luc mice. (Lower
figure) The schematic representation of the
neuronal routes how light resets adrenal or pineal hormones.
IML, intermediolateral cell collumn, PVN, paraventricular
nucleus of the hypothalamus, SCG, superior cervical ganglion,
SPvZ, subparaventricular zone.Upper figure is from Cell
Metabolism, 2, 297-307, 2005. |
|
|
Monitoring bioactive markers in the brain is fundamental
to clarify the circadian time-keeping system in the brain.
In collaborating with Professor Daiichiro
Nakahara, we
first devised a new method to monitor mammalian melatonin
in the cerebral ventricle by utilizing the lintracerebral
microdialysis probe. It is almost half the century since
the hormones such as melatonin and cortisol have the diurnal
rhythms. However, the analysis of dirunal secretion of
hormonesis hampered by that there are no system to record
continuously in a single body. We developed the microdialysis
system to analyze the melatonin secretion for several months.
By this method, we have revealed for the first time amine-dependent
and nerve-independent rhythms. This approach will became
more powerful when applied tot the genetically altered
mice. |
1) |
Nakahara D, Nakamura M,
Iigo M, Okamura H: Bimodal circadian secretion of
melatonin from the pineal gland in a living CBA mouse. Proc.
Natl. Acad. Sci. USA 100, 9584-9589,
2003. |
|
|
Fig. 10 A double plot
of melatonin levels and locomotor acivity for three mice
in LD and DD cycles. Note parallel change of melatonin
levels of locomotor activity in both LD and DD conditions.
Melatonin was measured from the cerebrospinal fluid
by the transverse microdialysis probe in the pineal
glands. From Proc. Natl. Acad. Sci. USA 100, 9584-9589,
2003. |
|
|
Finally we tried to monitor the gene
expression of clock gene st real time by inserting the
optical fiber directly to the SCN in the brain in freely
moving mice. We inserted an optical fiber just above
the SCN of mPer1-luc transgenic mice and succeeded to
record for several days oscillating luminescence that
accurately mirrored native mPer1 mRNA expression. By
this method, we first revealed mPer1 gene is activated
in the day time, and rest in the night time. These data
not only for the first time demonstrate a ticking biological
master clock in the brain of a living mammal, they also
show that real-time optical imaging of gene expression
is a new powerful tool to analyze the higher nervous
function of the brain including sleep/wake cycles(Nature,
2001). |
1) |
Yamaguchi S, Kobayashi
M, Mitsui S, Ishida Y, van der Horst GTJ, Suzuki
M, Shibata S, Okamura H: View of a mouse clock
gene ticking. Nature 409, 684, 2001. |
|
|
Fig. 11 (Upper
figure) Location of the
input end of the optical fiber in the mouse brain. (Lower
figure) Circadian fluctuation of luminescence in the SCN.
A mPer1-luc transgenic mouse, previously housed
under a 12h light/12h dark cycle, was continuously infused
with luciferin (10 µM in artificial cerebrospinal fluid) at
a rate of 15 µl/h. Luminescence was recorded under
constant dark conditions via an optical fiber positioned
above the SCN. One dot represents an average of the values
of 5 minutes. Hatched and closed bars at
the bottom of the figure represent subjective day and subjective
night, respectively.From Nature 409, 684, 2001, and Cell
Tiss Res 309, 47-56, 2002. |
|
|
Since Human Genome Project has finished,
the revolution of medicine is now going on: now all the
history of diseases and behavior of each person from
birth to death is referenced to its genome information.
However, even this type of correspondence studies develop,
there will be no progress how genomic information is
reflected to behavioral level. The functional significance
of circadian biology exists at this point: the unique
feature of circadian biology is that the gene transcription
occurs in the SCN reflects the behavioral and physiological
rhythms almost in a perfect state. This means that the
clock gene oscillation generated by the core loop in
each SCN neuron is coupled and amplified at the level
of the SCN neuronal nuclei. From here harmonized strongly
oscillating activities are spread into the whole brain
and to all those peripheral organs, which contain peripheral
clocks. As a final result, circadian changes are induced
in behavior and hormone secretion. Even though each neuron
in the SCN generates circadian oscillation, the system
of amplification and transmission needs to be well organized
to effectively transmit the time information to the peripheral
organs. The real time monitoring of clock gene oscillation
at gene, cell, tissue, brain, and system levels will
answer the question of how the time signal is born and
integrated to the upper layers of life. Investigations
of biological clocks open the fascinating perspective
to analyze the integrational mechanism of TIME in a vertical
arrangement, providing a bridge between single genes
and the living organism as a whole.
In May 2007, we moved from Kobe University to Kyoto University.
The new laboratory is Department of
Systems Biology in
the faculty of Pharmaceutical Science. Here, we want to
reset our work to clarify “How time
is generated and tuned”.
We hunt this esoteric secret poco a poco by believing a
fortune to Ride the Rhythm. |
|
Fig. 12 Integration mechanism of rhythm.
“Gene” depicts rhythmic transcription of mPer1 and mPer2.
“Cell” represents neuronal electrical activities of single
SCN neurons. “SCN” indicates the local neuronal and glial
circuits. “System” symbolizes behavior and hormonal secretion. |
|
|
|